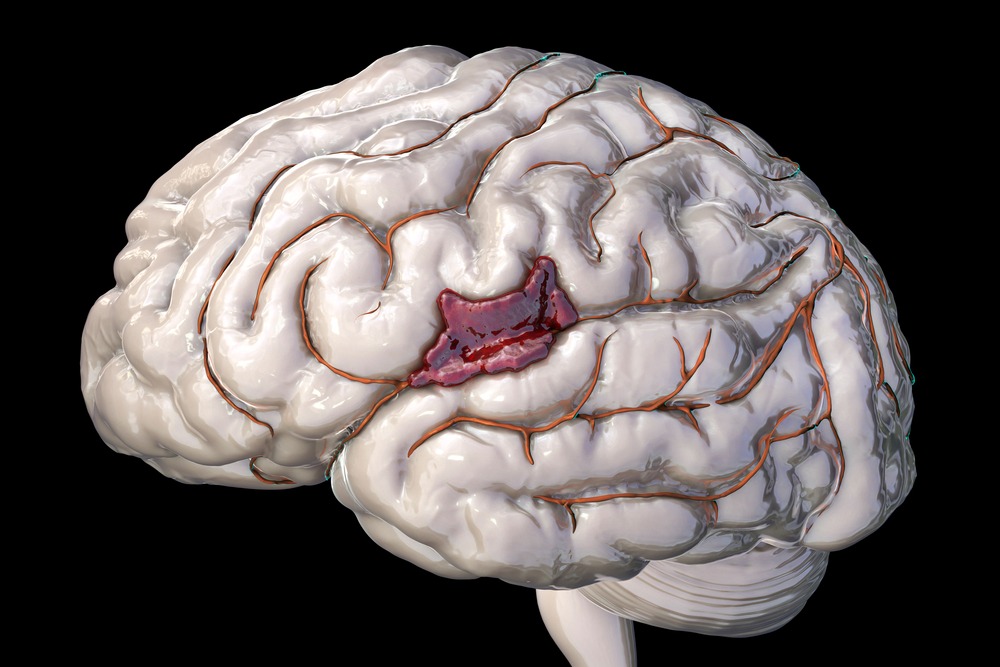
Neurological damage caused by traumatic brain injury (TBI) can vary dramatically, and so can the rate of repair that occurs naturally in human brains – but how this process occurs is not entirely clear to researchers.
Likewise, the reasons behind the wide variation of outcomes for treatment with neurological rehabilitation for injury or neurodegenerative disorders are a mystery.
Learning more about these mechanisms of brain repair could lead to treatments tailored to each individual patient, and that’s a reality that new research coming out of the state of Illinois in the US offers hope towards achieving.
Led by researchers at Northwestern University’s Shirley Ryan AbilityLab, a collaborative team including experts from the University of Illinois at Chicago developed a bioelectronic mini brain made of cortical spheroids to enable precise studies of how human brain circuits develop and repair themselves in vitro.
“The advances spurred by this research will offer a new frontier in the way we study and understand the brain,” said Shirley Ryan AbilityLab’s Dr. Colin Franz, co-lead author on the paper testing the technology.
“Now that the 3D platform has been developed and validated, we will be able to perform more targeted studies on our patients recovering from neurological injury or battling a neurodegenerative disease.”
According to the researchers, this is the first time the most sophisticated 3D bioelectronic systems have been combined with highly advanced 3D human neural cultures.
The study is the cover story for the March 19 issue of Science Advances.
How do the mini brains work?
The cortical spheroids used in the study were derived from human-induced pluripotent stem cells – a type of stem cell that can develop into any cell type in the body.
Leveraging a 3D neural interface system that the team developed, the scientists were able to create a mini laboratory in a dish specifically tailored to study the mini brains and collect different types of data simultaneously.
They incorporated electrodes to record electrical activity and added tiny heating elements to either keep the brain cultures warm or, in some cases, intentionally overheated them to stress them.
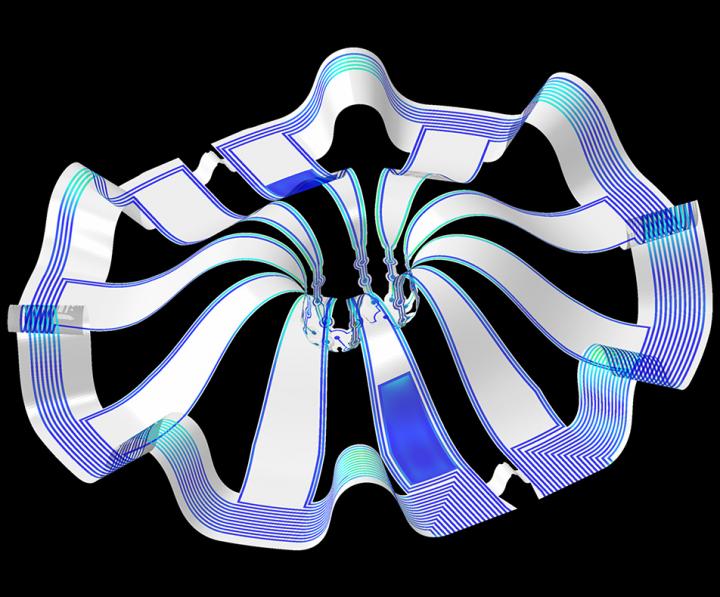
They also incorporated tiny probes, such as oxygen sensors and small LED lights to perform optogenetic experiments – a biological technique that involves the use of light to control neurons that have been genetically modified to express light-sensitive ion channels.
For instance, they introduced genes into the cells that allowed them to control the neural activity using different-coloured light pulses.
This platform enabled scientists to perform complex studies of human tissue without directly involving humans or performing invasive testing.
Northwestern University biomedical engineering professor John Rogers led the development of the cortical spheroids using technology similar to that found in phones and computers.
He said: “Now, with our small, soft 3D electronics, the capacity to build devices that mimic the complex biological shapes found in the human body is finally possible, providing a much more holistic understanding of a culture.
“We no longer have to compromise function to achieve the optimal form for interfacing with our biology.”
Potential clinical relevance to learning more about neurological injury and repair mechanisms
In theory, any person could donate a limited number of their cells (e.g., blood sample, skin biopsy).
Scientists can then reprogram these cells to produce a tiny brain spheroid that shares the person’s genetic identity.
The authors believe that, by combining this technology with a personalised medicine approach using human stem cell-derived brain cultures, they will be able to glean insights faster and generate better treatments.
“As scientists, our goal is to make laboratory research as clinically relevant as possible,” said Kristen Cotton, research assistant in Dr. Franz’s lab.
“This 3D platform opens the door to new experiments, discovery and scientific advances in regenerative neurorehabilitation medicine that have never been possible.”
Bioelectronic 3D neural interface technology could mimic other parts of the body
According to the researchers, current electrode arrays for tissue cultures are 2D, flat and unable to match the complex structural designs found throughout nature – such as those found in the human brain.
Even when a system is 3D, it is extremely challenging to incorporate more than one type of material into a small 3D structure.
With the advance from the team led by Northwestern University, however, an entire class of 3D bioelectronics devices has been tailored for the field of regenerative medicine.
Yoonseok Park, postdoctoral fellow at Northwestern University and co-lead author of the study, said: “This is just the beginning of an entirely new class of miniaturised, 3D bioelectronic systems that we can construct to expand the capacity of the regenerative medicine field.
“For example, our next generation of device will support the formation of even more complex neural circuits from brain to muscle, and increasingly dynamic tissues like a beating heart.”
As a next step, the scientists will use the devices to better understand neurological disease, test drugs and therapies that have clinical potential, and compare different patient-derived cell models.
It is hoped that this understanding will enable a better grasp of the individual differences that may account for the wide variation of outcomes seen in neurological rehabilitation.