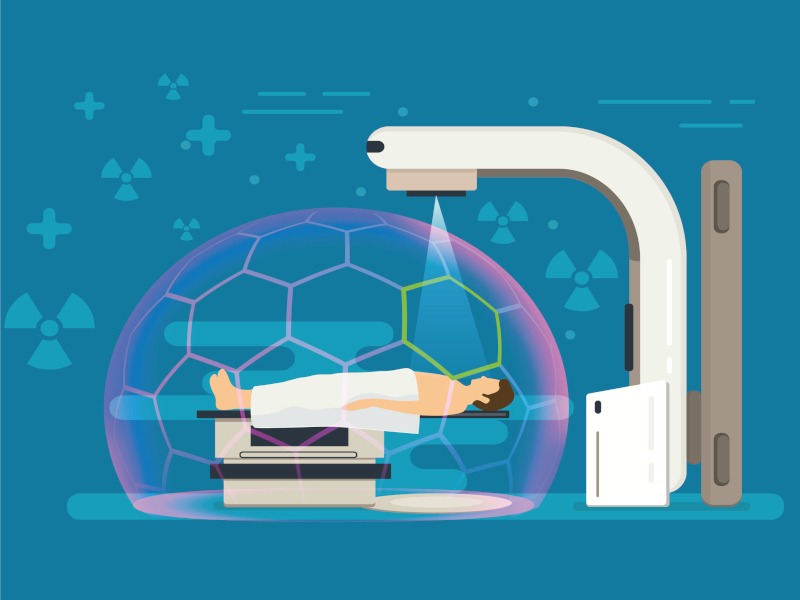
Radiotherapy, which involves directing high-energy radiation directly at a tumour to kill the cancer cells, is used in the treatment of about half of all cancer patients. A long-standing challenge has been to make sure that a maximum dose is delivered to the tumour, while sparing the surrounding tissue. The effect of damaging healthy tissue includes injury to the gastrointestinal tract, causing mucositis, which can cause ulcers, infection and increased mucus secretion. As well as being highly painful, oral mucositis can make it difficult to eat, having a detrimental effect on a patient’s quality of life.
Sometimes the consequences can be even more serious, depending on where the tumour is located. The clinician’s job, explains Dr James Robar, professor at Dalhousie University and chief of medical physics at Nova Scotia Health, is both to “wrap a very high radiation dose around a tumour” and to “control that radiation dose so it falls off to a very low level as quickly as possible outside the boundaries of that tumour”.
Keeping momentum in treatment
The location, size and shape of the tumour, which may be irregular, can all add to the difficulty in addressing the problem. There have, however, been developments that make it easier to avoid damage to healthy tissue. Radiation is now commonly delivered by linear accelerators, which approach that irregular tumour from different angles, using different beams. In doing so, they create a three-dimensional distribution of the radiation dose, matching the radiation beams very carefully to the shape of the tumour.
While this is generally highly effective in protecting the surrounding tissue, the success rate can drop in cases where the cancer is not localised. Patients with head and neck, oesophageal, gastrointestinal and rectal cancers are more likely to develop mucositis, with some stopping treatment as a result.
Another difficulty arises with the need to make sure the tumour itself receives the correct radiation dose. When radiation is directed at the top layers of the patient’s skin – for example, in post-mastectomy patients where the surgical scar is being treated, or in patients with head and neck cancers or malignant melanoma – the maximum dose typically occurs over a centimetre deep below the skin, rather than on the skin itself. In these cases, clinicians use a bolus: a device made with material with properties similar to those of human tissue, which is placed over the site of the tumour to make sure that the tumour itself receives the correct radiation dose. A bolus can also be used to modify a dose subdermally.
There are technical challenges to designing a bolus, however. As Robar points out, the surfaces are often “complex”, and sometimes they are “treating around surgical deficits, where surgery has been done previously”. One of the materials traditionally used to make a bolus is wax, which is inefficient and has limited accuracy, as well as being uncomfortable for the patient.
Creating devices tailored to the individual
The turning point came in 2013, says Robar, when many of the patents on 3D-printing technology expired. In 3D printing, or additive manufacturing, a digital image is used to create three-dimensional objects from the bottom up by adding a layer of material at a time. It is a particularly useful technology when an individual, tailored device is needed.
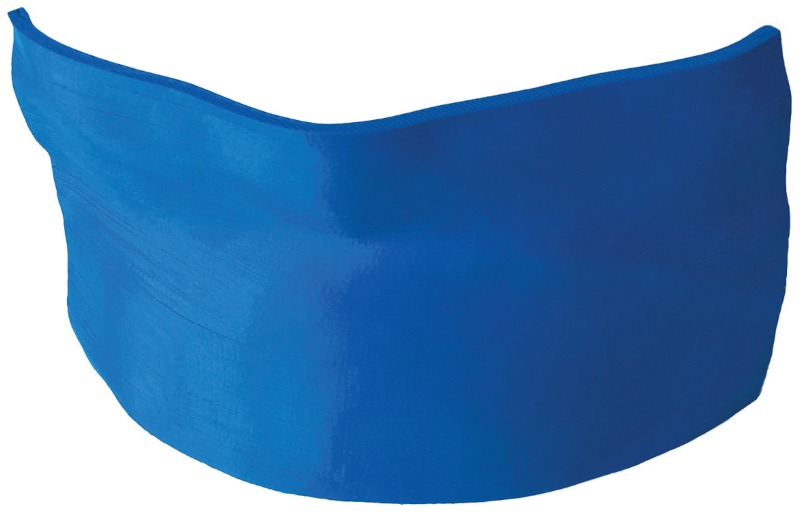
Researchers recognised the high-resolution CT scans that cancer patients undergo could also be used to create algorithms that would instruct a 3D printer to make a bolus tailored exactly to the anatomy of an individual patient.
This meant, as well as making sure the patient received the right dosage to the skin, the bolus could be shaped so it matched the shape of the tumour exactly. In a trial of 16 post-mastectomy patients, Robar and his colleagues found that the ability of the 3D-printed bolus to fit the patient was significantly improved and the air gaps diminished.
Adaptiiv, the company Robar set up with his colleagues to market the technology, has now partnered with HP to offer an on-demand service, whereby clinicians use the software to design the bolus in their clinic and then have it printed remotely and delivered three days later. Although Adaptiiv was one of the first companies to offer 3D-printed boluses, others have since followed suit.
“Researchers recognised that the high-resolution CT scans that cancer patients undergo could also be used to create algorithms that would instruct a 3D printer to make a bolus tailored exactly to the anatomy of an individual patient.”
Adaptiiv also markets Modulated Electron Bolus (MEB) software. While X-ray photons are normally used in radiotherapy, sometimes in the treatment of superficial tumours such as skin cancer, modulated electron radiation therapy (MERT) is used instead. Using an electron beam, says Robar, it is possible to “mirror the shape of the tumour with the shape of the 3D printed bolus to allow selective absorption of that electron beam. The end result is the distal or deep boundary of the radiation will match the boundary of the tumour quite closely.” This makes it possible to “spare several centimetres of healthy tissue at the deep aspect of the tumour, in some situations where you couldn’t before”.
A revolutionary treatment
A 2021 review of research on 3D-printed boluses described the technology as “revolutionary in superficial tumour radiotherapy.” It can “reduce the air gap, improve the accuracy and uniformity of dose, better protect normal tissues, and has clear advantages in cost and time efficiencies”. It acknowledged, however, that there was “not yet a consensus about the material choice, and frequency of application”.
Another option for minimising radiation dose to healthy tissues is to create protective shields (or masks). These are typically used for head or neck cancers and are designed to protect the oral cavity, tongue, gums and temporal region. It can, however, be challenging to create shields that conform to the patient’s particular anatomy – if, for example, a patient is missing some teeth. The traditional solution of casting several moulds is expensive, labour-intensive and uncomfortable for the patient.
“They will fit patients, they will optimise radiation dose distributions to patients, and it will provide a much more personalised approach.”
James Robar
Oral stents are also frequently used to keep the mouth open during radiotherapy to move the healthy tissue out of the way of radiation. A hydrogel rectal spacer, placed between the prostate and the rectum, is sometimes used to protect healthy tissue during radiotherapy to treat prostate cancer.
3D shields – the perfect solution?
The use of 3D printing enables clinicians to design a shield that perfectly matches a patient’s individual anatomy. These shields are quicker to create and involve no discomfort for the patient.
In developing 3D-printed shields, James Byrne, assistant professor of radiation oncology at the University of Iowa, and his colleagues spent some time testing different high atomic number (Z) materials to determine which would be most effective at attenuating the radiation dose. Lead proved particularly successful.
The team then used CT scans to create the 3D-printed shields and tested them on rodents to see how effective they were at shielding the oral and prostate tissue during radiation to the oral cavity. The results were impressive: all seven of the control rodents developed gross ulcerations on the tongue, while none of the rodents protected by the shield did. This experiment was followed by tests on pigs to see if the technique was scalable rather than to assess effectiveness.
They then carried out dosimetric modelling to assess how effectively the devices could protect healthy tissue. The modelling revealed that irradiation of oral mucosa in human patients could be reduced by 30% in those undergoing head and neck cancer radiotherapy and by 15% in patients with prostate cancer – without affecting the radiation dose to the tumour. The rectal radioprotectant device was more cost effective than a hydrogel rectal spacer. The technology is some way off being commercially available, but Byrne believes that the sums add up: “I think even though there may be a bigger upfront cost to having this device in place, if we’re able to reduce that degree of toxicity, then it’s going to significantly reduce the cost of the backend, or the care of some of these conditions afterwards.”
The potential of 3D printing in radiotherapy has not yet been exhausted. Robar sees possible uses for the technology in brachytherapy, in which radiation is delivered by a source placed inside the body, either via a catheter or an applicator, to treat a localised cancer. It is commonly used to treat gynaecological cancers, such as cancer of the cervix or endometrium. Standard gynaecological applicators, says Robar, are often “rudimentary”, and he believes that it will be possible to use 3D printing in combination with biocompatible materials to customise each applicator to the individual patient’s body. “They will fit patients, they will optimise radiation dose distributions to patients and it will provide a much more personalised approach,” he says.
Design to order
Around 50% of cancer patients will undergo radiotherapy as part of their treatment. During the procedure, patients are required to wear a bolus, but current off-the-shelf iterations leave spaces between the device and patient’s anatomy, which can result in cancerous cells receiving a lower dose than what’s necessary, as well as healthy tissue receiving a higher dose than what’s desirable. Medical personnel don’t always have to spend individualising each bolus to patient specifications, so 3D Systems developed an alternative 3D-printing workflow, which it calls VSP Bolus. The process works by clinicians submitting a patient’s CT scan data, which is segmented and used as a basis for a custom bolus designed via its DICOM To Print (D2P) software. Once ready, the device is sent for production at one of 3D Systems’ certified medical instrument manufacturing facilities. The technology arrived on the scene in April 2022 and is currently the only design and production service for a bolus based on a patient’s treatment plan.